Domain 1: Early stages of planet formation
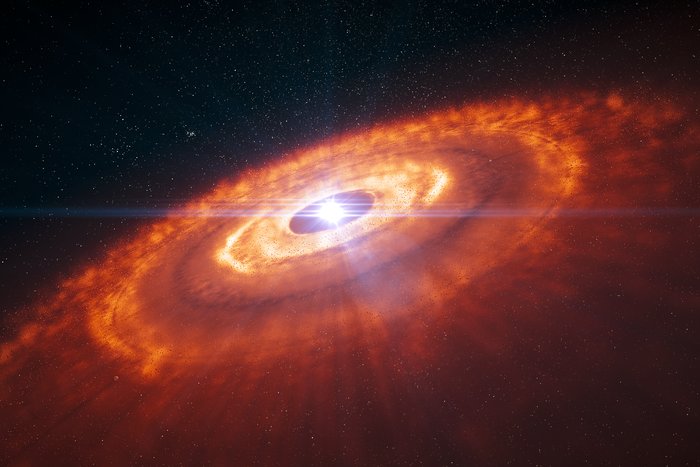
Credit: ESO/L. Calçada
Despite the thousands of new planets discovered over the past two decades, the detailed physical and chemical processes involved in the formation of planetary systems are still being debated. However, it is generally accepted that planet formation begins in a protoplanetary disc of gas and dust that assembles following the collapse of the parent molecular cloud core, leading to the birth of the central star. Various scenarios, as well as variants of the same scenario, have been proposed for the formation of both gas giants and rocky planets. In all cases, the first few million years of disc evolution represent a crucial phase setting the stage for planet formation. During this time, the disc is still gas dominated and its evolution is driven by mass and angular momentum transport mechanisms. Solids presents in protoplanetary discs, although minor in term of mass, are also important as they constitute the building blocks of future rocky planets and cores of giant planets. These solids suffer a variety of interactions with the gas phase, which have important consequences on their chemical, physical, and orbital parameters.
Among important mass and angular momentum transport mechanisms are MHD-induced turbulence and torques and/or fragmentation induced by gravitational instability, photo-evaporation, and two-fluid instabilities (such as the streaming instability) – all of which are potentialengines for pebble and planetesimal formation. Significant progress has been made in modelling such processes, e.g.: magneto-centrifugal winds proposed as an alternative to the conventional magneto-rotational instability (MRI) to drive angular momentum transport (Bai & Stone 2013); the complex interplay between radiation and self-gravity, possibly shaping early disc evolution (Rogers & Wadsley 2011; Galvagni et al. 2012); and radiation-hydrodynamic models of photo-evaporative winds and their observational signatures (Ercolano et al. 2015). Regarding the evolution of the coupled gas-solid system, first simulations capable of predicting the mass spectrum of planetesimals formed by streaming instability have been conducted (Schaefer et al. 2017). However, no model exists that combines two or more such mechanisms, and state-of-the-art streaming instability computations are still local rather than global. For instance, MHD turbulence in the presence of self-gravity is completely unexplored. More crucially, until recently, observations of protoplanetary discs have provided little evidence that any of these processes can take place. But over the last couple of years the new observational capabilities provided by ALMA and the progress in scattered light imaging techniques (for example with SPHERE) have begun to reveal a wealth of substructures in discs, including dust rings, spiral structures (Garufi et al. 2013; Benisty et al. 2015) and, perhaps, the first evidence of global modes induced by self-gravity (Perez et al. 2016). The new and upcoming disc observations are providing an unprecedented stream of new data that will finally provide quantitative constraints for models and simulations. Mock observations of disc simulations are just now starting to be used as new tools to quantify such constraints (Dong et al. 2015; Mayer et al. 2016). Finally, disc observations and models have not yet been significantly confronted with cosmochemical constraints from the meteoritic record in the Solar System, a major gap that needs to be filled. Specifically, formation models for chondrules, a ubiquitous component of chondritic meteorites, have focused on thermodynamical requirements of possible formation mechanisms (Boley et al. 2013; Stammler & Dullemond 2014) but have generally neglected to address their composition and isotopic heterogeneity, which carries information on mixing and transport processes in the early protosolar nebula.
The research in Domain 1 will combine multi-wavelength observations of protoplanetary discs in varying evolutionary stages and environments using ALMA and SPHERE with physically realistic hydrodynamical models of gas-dust mixtures. These models will be benchmarked against the results obtained from early solar system materials that provide insights into the early stages of the proto-solar nebula that gave rise to our own Solar System. The latter will be achieved by including a diverse range of cosmochemical constraints, most notably from meteorites containing the oldest compounds known to date in our Solar System, i.e., calcium-aluminium-rich inclusions (CAIs) and chondrules. Finally, laboratory experiments aimed at reproducing icy dust grains, an important constituent of solids in discs, will be used to improve our understanding of disc composition and to better interpret disc observations.
Project 1.1: Observational investigations of protoplanetary discs Project leader: Hans Martin Schmid (ETHZ)Planet formation in discs around young stars is an important process in extrasolar planet research because this phase defines to a large extend the chemical and physical properties of the planets and, if no later dynamical events occur, the architecture of the resulting planetary systems. This project aims at clarifying the complex planet formation processes using new high-resolution maps of protoplanetary discs in scattered light from polarimetric observations with SPHERE-VLT and as well as continuum and line-emission data from ALMA.
Over the past 5 years the “Star and Planet Formation” group at the Institute for Astronomy at ETHZ has played a leading role in protoplanetary disc research with polarimetric imaging using NACO–VLT (Quanz et al. 2011, 2012, 2013; Garufi et al. 2013, 2014, 2016; Avenhaus et al. 2014a,b; Thalmann et al. 2015, 2016) and in the development and use of the Zurich Imaging Polarimeter (Schmid et al. 2006) of the new SPHERE–VLT instrument.
The discs imaged in polarised light exhibit a wide variety of structures like gaps, rings, and spirals (see Figure P1.1), which could be the results of embedded forming planets, disc instabilities, or evaporation caused by the energetic radiation from the star. However, just considering our scattered light data yields ambiguous interpretations. Therefore, complementary information from other wavelength bands is required for a better understanding of disc structures. For SAO 206462 (Figure P1.1) we combined scattered light disc structures and ALMA maps and found that the inner cavity size is less extended for the small dust particles seen in polarized light compared to the cavity of the larger dust particles seen with ALMA. This effect may point to a planet just inside the inner rim of the disc, which introduces a gas pressure bump and an associated pile-up of the large, cm-sized, dust particles (Pinilla et al. 2012) as seen with ALMA. This region could be ideal for rapid growth of protoplanets by pebble accretion, as described by Lambrechts and Johansen (2012).
The disc of SAO 206462 is just one good example of the great diagnostic potential of a combined analysis of polarimetric images from SPHERE with ALMA continuum and line maps (e.g., like in van der Marel et al. 2016) for determining the gas and dust disc geometry at a phase when planets form. Combined analyses like this can provide important new constraints for planet formation scenarios.
More information
More information is available on our dedicated website.
Team
Project 1.2: Radiative multi-physics simulations of protoplanetary discs Project leader: Lucio Mayer (UZH)
Protoplanetary discs undergo significant changes during their first million years of evolution. This is the time span during which gas giant planets and the first rocky planetesimals should arise. Furthermore, several physical mechanisms have been proposed that drive transport of angular momentum, turbulence, and density enhancements in both gas and dust. However, simulations so far have been unable to model the interplay between these mechanisms, and they have often neglected the effect of radiation due to computational complexity. In phase I of the NCCR, we began tackling the challenge of modelling multiple processes simultaneously in discs, drawing a new scenario for the formation of pebbles and planetesimals from vorticitytriggered two-fluid turbulence (Surville et al. 2016; 2017), creating the first global simulations of GI discs that resolve the collapse of clumps down to the size of Jupiter (Szulagyi et al. 2016, Mayer et al 2016a,b, Mayer et al. in prep.) as well as the first simulations that can model the MRI in a self-gravitating disc (Deng et al. 2018, in prep.). However, radiative transfer (RT) is still missing in these calculations, even though it can dramatically impact the outcome of any disc process (Mayer et al. 2007). The main goal of Project 1.2 is to include RT in global MHD simulations of discs with self-gravity. RT models will be developed jointly with Project 3.1 (which considers RT in exoplanet atmospheres). The inclusion of RT will be crucial to compare the results of simulations with the new disc observations of Project 1.1 since the spectrophotometric properties of discs will ultimately depend on its interplay with gas dynamics. This is a timely objective since multiple RT solvers for our hydro-codes have been developed within the PASC Network “DIAPHANE” (Reed et al. 2017).
More information
More information is available on our dedicated website.
Team
Project 1.3: Environment and timing of chondrule formation Project leader: Ingo Leya (UBE)
A long-standing question in planetology is the formation of chondrules, which are a major constituent of primitive meteorites originating from the asteroid belt. Chondrules formed over a timespan of ca. 4 Myr (e.g., Krot et al. 2009). However, the environment of chondrule formation is still not properly understood and it is debated whether there is a time gap of ca. 1 Myr between CAI and chondrule formation (Bollard et al. 2017). The major goal of this project is to better constrain the environment of chondrule formation by combining noble gas data and cosmic ray tracks, both being produced by galactic and/or solar cosmic rays, with relative ages determined using the 26Al-26 Mg dating system. A major focus will be if the newly formed chondrules were exposed to solar and/or galactic cosmic rays that led to production of cosmogenic noble gases and cosmic ray tracks. Therefore, the detection of cosmogenic noble gas and/or cosmic ray tracks implies that chondrule formation took place in less shielded regions far above/below the mid-plane and/or close to gaps in the protoplanetary disc. Note that gaps in accretion discs seem to be common, as evidenced by recent ALMA results. In the next step, we exploit the expertise on 26Al-26 Mg dating obtained in the first period of this NCCR and further constrain the timing of chondrule formation in relation to their formation environment. Another focus will be whether there were several chondrule formation environments and, if so, whether they were distinct in time and/or space in the disc. To this end, we will determine 26Al-26 Mg ages for selected primitive chondrules for which parts have been studied previously for cosmic ray tracks and noble gases. Where sample size permits, we will also determine 26Al-26 Mg relative ages for the chondrules studied for isotope anomalies in Project 1.4 and 1.5.
More information
More information is available on our dedicated website.
Team
Project 1.4: Building planetesimals - protoplanetary disc evolution traced by nucleosynthetic isotope variations in meteorites Project leader: Maria Schönbächler(ETHZ)
The goal of this project is to characterise the distribution and variability of nucleosynthetic isotope variations in chondrules, inherited from the precursor material and distinctly sampled by asteroids. To this end, we will separate chondrules from different primitive chondrites and analyse them for nucleosynthetic Ti and Cr isotope variations. This data will assess (1) the extent of transport and mixing in the region of the disc from which chondrites accreted and (2) the origin of chondrules. For the latter, the data will be combined with numerical simulations of chondrule formation (i.e., models for planetary bow shocks, shock induced by massive planets and spiral shocks in GI discs), which are developed in collaboration with Project 1.2 (postdoc position). The project also connects to Project 1.1, which will provide observational evidence for disc substructure associated with spiral shocks or planet-induced shocks using ALMA, and is complementary to Project 1.3 (timing of chondrule formation) and Project 1.5, which focus on investigating the chondrule-matrix complementarity.
More information
More information is available on our dedicated website.
Team
Project 1.5: The matrix-chondrule complementarity: constraints on the chondrule formation processes Project leader: Klaus Mezger (UBE)
Chondrites are the most primitive rock samples from the Solar System available for direct study in the laboratory. Physical models have been proposed that suggest chondrule formation is due to collision of primitive or partially differentiated planetesimals (e.g., Krot et al. 2005; Fedkin et al. 2012), while in contrast models based on element and isotope abundances would be more consistent with a process involving flash-heating and melting of dust aggregates in the disc (e.g., Morris & Desch 2010). Recently it has been suggested that a combination of the two models may account for petrographic features observed in some chondrules (Libourel & Chaussidon 2011). All these models need to satisfy the chemical and mineralogical observations made on chondrites and their different components. A key parameter is the admittedly debated observation of different, but complementary, major refractory element compositions of matrix and chondrules, leading to very similar bulk compositions within one chondrite group, albeit with possible different abundances of chondrules with different compositions (e.g., Hezel and Palme 2010). Recently it has been shown that this matrix-chondrule complementarity also applies to the siderophile isotopes of W and Mo in a CV meteorite (Budde et al., 2016a,b). It has also been shown that some isotopes show distinct differences bebetween chondrite groups (particularly 54 Cr, 50 Ti, Trinquier et al. 2009). Altogether these data suggest that different regions in the early solar system had distinct isotope compositions, but the reasons for these differences remains enigmatic. By studying the stable isotope compositions of separated matrix and adjacent (ideally) individual chondrules of various types of chondrites, we will evaluate chemical differentiation and possible (un)mixing processes during chondrule formation and subsequent chondrite formation. The goal will be to obtain key information on the possible mechanisms of chondrule formation as well as on the physical state and chemical evolution of the solar nebula during the time interval of chondrite formation:
- For which element is there isotope variability between matrix and chondrules?
- Which proposed chondrule formation model can be reconciled with the isotope data?
- Is the chondrule forming process triggered by planetesimal formation?
- Is there evidence for a late injection of material from a nearby supernova during or after the time interval of chondrule formation and chondrite accretion?
More information
More information is available on our dedicated website.
Team
Project 1.6: Experimental study of the fate of volatiles in protoplanetary discs Project leader: Antoine Pommerol (UBE)
We propose to perform laboratory experiments that will provide new constraints for the processes controlling the association and segregation of volatiles and refractory material in the early phases of protoplanetary discs. These constraints will be used to improve the quantitative analysis of disc observations and will be important inputs for models of disc evolution. LOSSy, developed during phase I of this NCCR (Pommerol et al. 2015), focuses on the production of icy analogues of planetary/cometary material, their characterisation and their use in laboratory simulations. Our initial goal was the measurements of the visible reflectance properties of the samples to help interpreting data obtained by various solar system probes. The reflectance of the samples is characterised as a function of measurement geometry, wavelength, or polarisation. Recently, we have broadened the scope of our investigations to encompass the radio, microwave, and infrared spectral ranges and to study the effect of the samples irradiation by energetic particles. We now propose to build upon the existing expertise and infrastructure to apply our methods to the case of protoplanetary discs.
More information
More information is available on our dedicated website.
Team
Associate
Project: 1.7 Isotope astrochemistry: linking star formation with the solar system record Project leader: S. Wampfler, UBE
Description will follow
More information
More information is available on our dedicated website.
Team
Project 1.8: The Heliosphere and the dust characterisation the solar and interstellar neighborhood Project leader: V. Sterken, ETHZ
Description will follow
More information
More information is available on our dedicated website.
Team
Project 1.9: The role of circumplanetary disks in planet and moon-formation Project leader: J. Szulagyi, ETHZ
Description will follow
More information
More information is available on our dedicated website.
Team