Hydrodynamical simulations of protoplanetary disks and planet formation
Prof. Lucio Mayer
Introduction
Understanding the formation of planets requires first to understand the origin and development of their natal places, namely protoplanetary disks around young stars. From observational evidence disks are known to evolve through various phases in which several complex processes take place, regulating mass transport, thermodynamics and the gathering of gaseous and solid matter into planets. Among these processes, self-gravity, radiation transport, turbulence, magnetohydrodynamical instabilities, tidal effects and photoionizing radiation from massive nearby stars, disk-planet interaction in the form of tidal torques, all play a role. Furthermore, the disk itself forms from the turbulent collapse of a molecular cloud core, a process which is also highly complex and poorly understood. The range of scales involved is also huge, from pc scales to a fraction of astronomical unit. The wide range of scales and physical complexity make it mandatory to tackle these problems with 3D radiation hydrodynamical simulations incorporating possibly also self-gravity and magnetic fields. So far, simulations have a restricted dynamic range and have not included one or more of the key physical processes, such as self-gravity or MHD. With our numerical laboratory we will design the new state of the art simulations of disk formation and evolution, including all the relevant physics and connecting the relevant scales. State-of-the-art hydro codes such as GhaNGa and RAMSES-RT will be used, on the largest parallel supercomputers available in Switzerland, such as at the Swiss National Supercomputing Center, as well as abroad.
1 – Disk Formation and Evolution with realistic physics
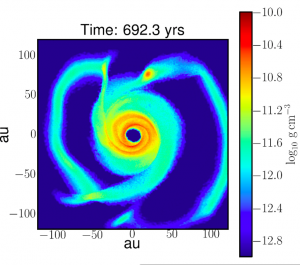
Logarithmic density map of a massive extended self-gravitating protoplanetary disk whose evolution has been computed with the 3D SPH GASOLINE code employing flux-limited diffusion combined with a photospheric cooling model (the model is described in Rogers & Wadsley 2011) . Jupiter-sized gravitationally bound clumps are forming along the spiral arms.
In order to understand the composition, structure and thermodynamics of protoplanetary disks it is necessary to understand how they assemble from the parent molecular core since crucial parameters such as angular momentum, temperature and mass of the disk are determined by the conditions accompanying the collapse of the core into a rotationally supported disk. Magnetic fields also play a crucial role as they cause, for example, dissipation of angular momentum. Initially the disk is very massive as the protostar is beginning to form, later mass will be accreted onto the star or dispersed by eg photoevaporation. The high initial mass implies the disk will be self-gravitating and, possibly, may enter a phase of gravitational instability.Ideally one would want a calculation that computes the first million years of disk evolution, up to the T-Tauri stage, starting ab initio. Performing one of such ab initio disk evolution calculations is currently prohibitive, even without radiative transfer, due to the huge range of densities and timescales. Achieving the ability to perform one of such calculations for the first time is one of the long term goals of PlanetS. With ChaNGa, indeed, we have the best tool available in the world as we can run efficiently on several thousand cores on large supercomputers. However, we will first proceed by performing separately (I) ab initio calculations of the first 105 yr of evolution with realistic physics, including radiative transfer and (II) very high resolution simulations of realistic disk models with self-gravity, radiative transfer, later incorporating MHD. The first calculations extend efforts done by us and other groups to understand the early stages of disks, which are becoming increasingly accessible with new instruments such as ALMA. The second type of runs will allow us to understand the effect of self-gravity in combination with realistic radiation physics as well as to explore the interplay with important physical effects triggered by the magnetic field, such as the MRI instability. Both gravitational torques exerted by spiral waves naturally arising in a self-gravitating disk and MRI-driven turbulent torques are known to extract angular momentum and lead to inward mass transport in the disk, which is crucial for disk evolution and the growth of the star. Furthermore, if the disk becomes gravitationally unstable it can fragment and form sub-stellar clumps, with masses comparable to gas giant planets or brown dwarf. This “disk instability” pathway to planet formation is still debated since fragmentation depends heavily on radiation transport, convection, and possibly would be affected by the development of MRI. 3D simulations incorporating the full inventory of physical processes will be designed for the first time in the context of PlanetS. In Figure 1 we can see a density map of a ragmenting disk forming gas giant-sized clumps in a simulation run with GASOLINE using the flux-limited diffusion method for RT.
2 – Formation and collapse of protoplanets by gravitational instability and other mechanisms
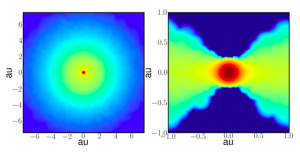
Close-up view of density structure of a ~ 3 Jupiter mass clump collapsed down to densities approaching the mean density of Jupiter (a few Jupiter radii in size), from Galvagni et al. (2012). In this case the clump was extracted from a global 3D disk simulation in order to be able to follow its collapse with enough resolution. The right panal is a zoomed-in version of the left panel.
The simulations of disk evolution cannot reach the resolution necessary to study the formation of planetary-sized condensations resulting from disk instability or other mechanisms. As part of the PlanetS endeavour we will develop the first simulations capable of resolving very local processes, such as gravitational collapse into protoplanets or the formation of vortices with arrange of scales that can accumulate dust particles and quickly grown them int planetesmals (Surville et al., in prep.). while computing the global disk evolution for extended time. This will be possible by exploiting the superior parallel scaling capabilities of the ChaNGa hydro code. This way we will merge the disk evolution simulations with the pioneering simulations of Galvagni et al. (2012), which have been the first to compute the 3D collapse of a protoplanetary gas clump with enough resolution to capture condensation the size of Jupiter, but had to give up on modelling the global disk flow and the concurrent orbital motion of the protoplanets in the disk. These simulations showed the clumps are disky and undergo similar processes as protostars during the collapse phase, such as becoming bar unstable, which accelerates the collapse by redistributing angular momentum on the orbital timescale. The collapse times were found to be at least one order of magnitude shorter than in previous analytical approaches that treat the clumps as collapsing spheres, pointing to a much higher survivability against tidal mass loss and planet migration into the star.
The planed simulations will include also H2 dissociation as the planetary core collapses to very high density and temperatures larger than 2000 K. The disk-protoplanet interaction (migration) will be naturally included. Semi-analytical models, in which the timescales and key features of these different processes can be easily incorporated and their effect tested in a statistical sense eg Galvagni & Mayer 2014) will complement the new simulations effort, being calibrated by them.